Rock Envy
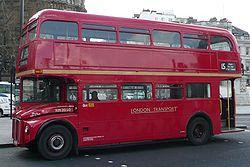
With the exception of Hayabusa, all asteroid missions to-date have been to targets bigger than 1 km in "spherical radius". Date Encounter Asteroid Radius (km) Spacecraft 1991 Flyby 951 Gaspra 6.1 Galileo 1993 Flyby 243 Ida 15.7 Galileo 1999 Flyby 9969 Braille ~1 Deep Space 1 2000 Flyby 2685 Masursky ~8 Cassini 2001 Landing 433 Eros 8.42 NEAR Shoemaker 2002 Flyby 5535 Annefrank 2.4 Stardust 2005 Sample Return 25143 Itokawa 0.165 Hayabusa 2006 Flyby 132524 APL ~1.1 New Horizons 2008 Flyby 2867 Šteins ~2.8 Rosetta 2010 Flyby 21 Lutetia 95.8 Rosetta This has led a number of people to express dismay that all the asteroids which have been identified for human exploration missions have significantly smaller estimated sizes. Date Asteroid Radius (m) 2016 2008 HU4 ~5 2017 1991 VG ~45 2019 2008 EA9 ~6 2020 2007 UN12 ~4 2025 1999 AO10 ~35 2026 2008 JL24 ~2.5 2028 2006 RH120 ~2.5 2029 2000 SG344 ~22.5 Notice that the scale has changed from km to m. Of course...